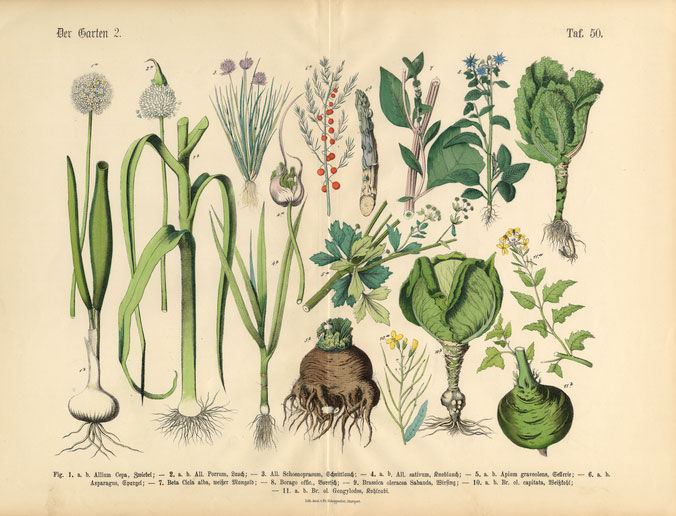
I've found politics so incredibly bleak lately, and I've been so frustrated by my inability to make any kind of difference whatsoever--for example, regarding the genocide in Gaza, arguably the worst crime of the 21st century (worse even than Bush's invasion of Iraq)--that I haven't written anything. I've retreated into other interests, such as science and philosophy. I hope to return to politics fairly soon, but I've needed a break.
I recently read a book as far removed from politics as possible: Brian Capon’s Botany for Gardeners (2005). I know almost nothing about botany, so I thought I might as well educate myself. I'm not exactly a gardener, but you don’t have to be a gardener to appreciate the book.
I know the subject of botany seems pretty random, but in case anyone is curious about the world of plants, here are some notes I took while reading...
***
Random bits of interesting trivia: How is it that sun-loving plants can bend and grow toward the source of light? Answer: when the stem is illuminated from directly above, “cells [in the stem] undergo equal rates of elongation, resulting in vertical growth. But when lit from one side, stems change direction because cells on the shaded side grow faster than those toward the light.” Their faster growth, of course, causes the stem to bend towards the more slowly growing side (the illuminated side). Fascinating! But why do shaded cells grow faster? Because of the hormone auxin. “When light strikes one side of a stem, auxin accumulates in the shaded side, causing the cells there to grow at the fastest rate.”
Similarly, suppose you tip over a potted plant so that the plant is horizontal now. You’ll soon find that the stem returns to its vertical growth. How? Because “under the influence of gravity, auxin collects in the lower side of the stem where it stimulates the cells to grow more rapidly than those across the top.” So the stem bends upwards.
What about the opening and closing of flowers? This, too, is controlled by hormones. When a flower opens, “cell expansion occurs in the petal’s upper surface; when the flower closes, cells in the lower petal surface increase in size.” The same principle applies in the case of Venus fly traps: their closing is just an exceptionally rapid growth response (on the part of cells on the outside). Pretty amazing!
The aging process in fruits and leaves is, naturally, directed by hormones, particularly ethylene:
When fruits ripen, various biochemical events take place. Color changes result from the breakdown of green chlorophyll and an increase in yellow, orange, and red pigments. Tannins, which protect immature fruits against predators, give way to sugars—making some fruits attractive to seed-dispersing animals. And, primarily as a result of ethylene’s influence, drastic changes occur in cell structure, including the breakdown of membranes and the softening of cell walls. The outcome of such processes is seen in the rapid deterioration of a fruit as it becomes overripe, ready to release its seeds. Unprotected by tannins, well-stocked with carbohydrates, and the cuticle layer degraded, the ripe fruit also becomes a perfect medium on which fungi can grow. Their presence eventually leads to the fruit’s decomposition.
…Leaf senescence [in autumn], prior to abscission [separation from the stem], includes the breakdown of chlorophyll and weakening of cell walls at the base of the petiole, in a narrow band of cells called the abscission zone. In spring and summer, auxin produced in the leaf keeps the abscission zone intact. But low night temperatures and short days in autumn cue the leaves to reduce auxin production and increase the liberation of ethylene. The latter stimulates an enzymatic breakdown of cellulose walls and pectin in the middle lamellae—the glue holding cells together. The restriction of ethylene’s destructive effects only to cells in the abscission zone illustrates the precise control plants exercise over their hormone systems.
Let’s turn to the question of how the plant uptakes water. It starts with osmosis into the root. “During osmosis, water molecules attempt to equalize their concentration on both sides of cell membranes when they move into or out of living protoplasm [i.e., the interior of the cell].” In brief, here’s how it works:
In most soils, small quantities of salts are dissolved in large volumes of water. Conversely, the protoplasm of epidermal cells [in roots] contains lesser amounts of water in which salts, sugars, and other substances are concentrated. Thus, when water moves (diffuses) from the soil, where it is most abundant, it seeks to dilute the cells’ solutions.
When water enters the cell it’s stored in the large central vacuole, which expands and presses the cytoplasm against the rigid cell wall. Eventually the cell becomes turgid, or fully inflated. “Cell turgor gives firmness to water-filled tissues. The difference between crisp and wilted lettuce leaves illustrates the nature of turgid and nonturgid (flaccid) cells.” I hadn’t known that wilted plants are literally just…their cells aren’t fat with water.
So how does the water get up to the stem and leaves? Basically, the root’s turgid epidermal cells discharge water into spaces between other cells, cortex cells, where the water works its way into the hollow tubular cells of the xylem at the root’s center. Articles online have technical details, but the upshot is that water is pushed up the xylem with a slight pressure. At the same time, the evaporation of water from the surfaces of cells in the leaves generates a “pulling” force that can lift water hundreds of feet up a tree.
Okay, let’s move on to the main attraction: photosynthesis. It’s absurd I’ve never known exactly what photosynthesis involves. After all, it’s “the single most important process on Earth,” since “the large-scale storage of abundant sunlight in the form of compact, energy-rich food molecules is the unique function of Earth’s flora.” In fact, “autotrophic [i.e., making their own food] plants hold the key to life on Earth; they alone are the intermediaries between the sun and all other creatures. And it is their leaves’ microscopic chloroplasts that have the awesome responsibility of making the system work.” So let’s investigate photosynthesis, the process that makes it possible for you and me to live.
(It’s worth noting that photosynthesis provides, directly and indirectly, not only our food and oxygen but even most of the world’s energy, almost 90 percent of it, through fossilized photosynthetic fuels. It’s also, apparently, the sole source of the organic carbon that all carbon-based life forms use to build themselves.)
Combining the book and a lot of articles, I’ve arrived at this understanding: photosynthesis takes place in the organelles called chloroplasts, which contain chlorophyll molecules (two different kinds: chlorophyll a and b). They also have carotene, which reflects orange/red/yellow light (unlike chlorophyll, which reflects the green wavelength), and xanthophyll, which reflects yellow. By the way, “when leaves turn yellow [in autumn], they simply lose chlorophyll that had previously masked the appearance of the orange and yellow carotene and xanthophylls.” Interesting! The point of photosynthesis is that the wavelengths of light absorbed by these molecules are transferred into the synthesis of food, in other words carbohydrates (sugar and starch), fats, and proteins.
It might be obvious, but it’s interesting to reflect that what a leaf is, in essence, is just a “solar collector crammed full of photosynthetic cells.” Actually, that’s too simple: leaves also contain thousands of pores on their surface (mostly their bottom surface) called stomata, through which carbon dioxide enters and oxygen is released. Anyway, leaves exist to produce the plant’s food, from light, water, and carbon dioxide.
The way it works is…insanely complex. Of course. But I’ll try to make it comprehensible. First, chlorophyll b, carotene, and xanthophylls absorb and channel photons to chlorophyll a, whose electrons are thereby boosted to a higher energy potential. In their energized state, chlorophyll’s electrons are diverted into an “electron transport chain” that ends in the formation of the molecules NADPH and ATP. Meanwhile, chlorophylls get back the electrons they’ve lost by the splitting of water molecules (so that these molecules donate electrons to the chlorophyll), which breaks down water into its components hydrogen and oxygen. In its new gaseous form O2, oxygen then flees the hectic scene into the atmosphere.
In the second stage of photosynthesis, carbon dioxide links to sugar molecules called ribulose bisphosphate, producing new molecules that then, with the help of ATP and NADPH, are rearranged to form, ultimately, glucose and other sugars, including more molecules of ribulose bisphosphate (thus allowing this stage of photosynthesis to keep repeating again and again as long as ATP and NADPH are available). So we’ve finally arrived at carbohydrates that the plant can use for energy! Specifically, glucose, fructose, sucrose, starch, and cellulose. Yay! These sugars can also be used to form lipids and amino acids (i.e. proteins). Starch (made of glucose) is the principal food stored in plant cells, while cellulose (also made of glucose) is incorporated into the structure of cell walls.
Okay, enough about photosynthesis. My brain hurts. Just to write those four paragraphs I had to spend hours trying to piece together the story from a dozen articles and a couple books.
Let’s move on to reproduction and flowers. Plants produce two kinds of reproductive cells: gametes and spores, the latter of which grow into a plant simply by mitotic cell divisions. Only the more primitive plants, like ferns, rely on spores. More interesting is sexual reproduction. The whole point of flowers, of course, is to attract insects and other small animals so that they’ll propagate pollen, each grain of which contains sperm. Stamens are the male reproductive structures, which consist of a filament and an anther on its top. Within the anther is where pollen develops. Pistils, the female structures, are divided into three parts: at the top is a stigma, a sticky receptive surface to which pollen adheres; the long style elevates the stigma to a favorable position for pollen collection; and at the base is an ovary. The ovary is what ultimately becomes a fruit. As a reward for their assistance with reproduction, animals are given a nutritious liquid called nectar at the base of the petals, pistil, and stamen. (When they’re maneuvering around to access the nectar, pollen is scraped off them and new pollen brushes against them. “The petal’s color patterns, called nectar guides, are virtual road maps directing visiting insects to the sweet food, past waiting stamens and pistils.”)
Wind and water can also pollinate plants. In grasses, for instance, “the anthers dangle on quivering filaments,” and vast amounts of pollen are borne on breezes so that hopefully some tiny fraction will find its way to appropriate flowers. The most ancient pollen producers are gymnosperms, non-flowering seed-producing plants, whose seeds aren’t enclosed in an ovary or flower. (The opposite type, the flowering type, is called angiosperms.) Their pollen is scattered, frequently, from male cones—hence the name conifer trees—which are actually just modified leaves. This system evolved before insects and hasn’t changed since, so it has no need of flowers to attract animals. Some flowering plants, on the other hand, are aquatic species that use water to disseminate pollen. For example, the underwater plant called ribbon weed forms female flowers on long stalks that reach to the water’s surface, whereas male flowers, formed near the base of the plant, simply float to and on the surface like tiny sailboats. “Propelled by gentle winds, some [of these little sailboats] eventually make contact with the female flowers and transfer pollen.”
(By the way, bamboo “trees” are actually grass! This is because their stems are hollow and don’t have the same vascular system as trees. In lawn grass, what is most visible is not the stem but the long, thin leaves that jut up from the stem. Even lawn grass grows tiny flowers—if you don’t mow it.)
Many species of flowering plants have evolved means of ensuring cross-pollination, which is more advantageous than self-pollination. These means include “self-incompatibility, chemical barriers in the stigma that treat a plant’s own pollen as if it were from another species; spatial separation of the anthers and stigmas in a bisexual flower; or staggered timing of pollen release and the stigma’s receptiveness in each flower.” Cross-pollination also happens when separate male flowers (staminate) and female flowers (pistillate) form on the same plant.
Here’s something I didn’t know: the reason many flowers close at night and on rainy or overcast days is to protect the pollen from moisture.
A couple of useful paragraphs:
The stage is set for reproduction when, by one means or another, compatible pollen comes to rest on a flower’s stigma. Of the two cells within a pollen grain, one is destined to grow into a long tube, a pollen tube, that penetrates the pistil’s tissues in search of a microscopic opening in one of the ovules, located in the ovary. Germination and growth of the pollen tube is rapid and is promoted by food substances and hormones supplied by the stigma and style. Botanists still don’t understand how a pollen tube locates the ovule’s tiny pore…
The second of a pollen’s cells divides to become two sperm that move through the pollen tube and enter the ovule. Before the pollen tube and sperm’s arrival, each ovule must be equipped with an egg, ready for immediate fertilization. A zygote is formed when one of the two sperms unites with the egg. The second sperm combines with another cell in the ovule. The product of that union is a temporary food-storage tissue, called the endosperm, used to nourish the zygote as it grows into an embryo, the miniature plant within a seed…
As ovules grow and mature into seeds, they remain enclosed by the ovary, which slowly enlarges to become a fruit…
Some fruits, such as peaches, apricots, plums, and cherries, have only one seed in their hard center; strawberries, on the other hand, have a whole lot of tiny, ripened ovaries (the true fruit) on their surface, each with a seed in it. Botanists have classified fruits into several categories. For example, “a simple fruit is derived from a flower bearing a single ovary; examples include tomato, orange, grape, melon, and peach. An aggregate fruit comes from a flower having many ovaries. Thus, an aggregate fruit is a collection of small fruits borne on a single receptacle, such as blackberry, raspberry, and strawberry.” The fruit’s function is to protect the seed until it’s ready for dispersal. One method of dispersal is to be eaten by animals (the hard seeds pass through digestive tracts without being harmed). Another method is exemplified by dandelions, whose small fruits have feathery parachutes that can disperse them through air. (A botanist’s definition of fruit, by the way, is obviously different from the popular definition of fruit as being something edible and sweet. You wouldn’t want to eat dandelion fruits. Likewise, in the botanical sense, tomatoes, bell peppers, cucumbers, peas, okra, olives, avocadoes, corn, green beans, chick peas, nuts, etc. are all fruits, since they come from the flowering part of a plant and contain seeds. Vegetables are the other edible parts of a plant, like the leaves, stem, roots, and bulbs.)
The book’s last chapter, on genetics and inheritance, is confusing as hell, being much too cursory. Sporophytes, gametophytes, megaspores, alternation of generations…and genetics is complicated enough even without these weird alternating generations. So screw it, I’m done with botany for now. At least I know a little more than I used to.
Oh the miracles and beauties of nature! And oh, the headaches one gets when reading about them!
(But at least they're not as harmful to mental health as the headaches one gets when reading about politics.)
Comments